In his fabulous nonsense poem, The Walrus and the Carpenter (1871), Lewis Carroll groups cabbages and kings together. Upon reflection, we might ask what cabbages and kings have in common. Probably nothing.
Nevertheless, there are some cases in nature where similar groupings might call for a different answer. Let me riddle you this: what do marine cone snails have in common with a tall tree growing in tropical Australia? And what do sperm whales have in common with a desert shrub?
Don’t be quick to confidently reply “nothing”! The true answer is, “You would be surprised!”
Toxic tree and savage snail
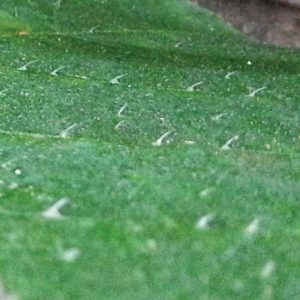
The Australian stinging tree’s stems and leaves are covered with longish hairs a quarter inch long in a layer so thick it looks like velvet. Picture by Norbert Fischer and used under a Creative Commons Attribution-Share Alike 4.0 International license.
The tree in question is the Australian stinging tree Dendrocnide excelsa which grows 35 m (115 ft) tall. Its stems and leaves are covered with longish hairs 1/4 inch long in a layer so thick it looks like velvet. But looks can be deceiving. These hairs are actually hollow tapering tubes with a small bulb at the tip. If anyone or anything happens to brush one or more of these trichomes/hairs, the victim receives an excruciatingly painful sting which can cause symptoms that last for days or even weeks.
There are two features of this event that interest us, the delivery of the sting, and the nature of the poison.
The sting mechanism is certainly interesting. According to a recent article, the needle-shaped hairs (trichomes) “act as hypodermic needles that, upon contact with skin, inject specific pharmacological mediators contained within the trichome fluid…”1
A leave that injects? That might seem a bit far-fetched. After all, how can a hollow tube inject anything? To answer that, a different study points out that it all comes from the complex design of the trichome (hair).
Except for a flexible base, the rest of the hair is a hollow tube whose walls are made very stiff with calcium carbonate and silica. The interior of the hollow tube is filled with a cocktail of nasty compounds. The scene is set for the following event:
“The stinging cells are essentially hollow from the base to the bulbous tip and break off with the slightest touch. Breakage creates a sharp edge connected to a large liquid reservoir similar to a hypodermic needle. Pressure applied to the trichome [the hair] will compress the bladder-like base and eject the irritant fluid from the tip in an action analogous to the plunger in a hypodermic syringe.”2
Concerning that process, the authors of that paper declare: “Stinging hairs – even as mechanical structures – are not simple cells with mineralized walls, but stunning examples of unique plant microengineering.”3 That certainly sounds like design!
The Australian stinging tree is classified in the same plant family as common stinging nettles. The nettle characteristics are very similar to the tree except for size (nettles are much smaller), and the nature of the irritant, which is not dangerous in the case of the nettles.
Sinister similarity
But finally getting back to our riddle, we now discover that the mode of delivery of the nasty chemicals in the tree (and the nettles) is very similar to what we see in some animals such as poisonous spiders and marine cone snails. Cone snails are dangerous predators that we see in tropical seas. Up to 22 cm or 9 inches long, these creatures hunt worms, other mollusks, or fish. Some of the 500 species exhibit toxin so potent that it can kill people. Interestingly, these nasty cone snails inject the poison into their victims by a syringe-like action similar to that of the stinging tree.
However, it is in the appearance and action of the poisons that the similarity between stinging trees and cone snails becomes particularly clear. As a recent article declares:
“Our results provide an intriguing example of inter-kingdom convergent evolution of animal and plant venoms with shared modes of delivery [as we have seen], molecular structure, and pharmacology.”4
Translating this into ordinary English, they are telling us that the poisons produced by the stinging tree and the cone snail are very similar to each other. The term “convergence” communicates the idea that these highly unusual products come from totally different sources. How the tree and the marine snail might have obtained these products through an evolutionary process, is unknown. Hence the term convergence suggests that organisms converged on the same obscure choice for unknown reasons by unknown processes. Despite the obscurity of the explanation, most scientists are sure that there must be an evolutionary explanation.
The most remarkable aspect of the unexpected similarity between a tree and a marine snail is in the nature of the poisons that they produce. From the variety of compounds in the venomous liquids, the team found that the most effective products in the tree were “mini proteins” of only 36 amino acids long. Despite the fact the molecule is so short, the order of amino acids is unlike any other protein known in any other organism. Because the molecules are so unique to the stinging tree, the scientists called them gympietides (after the name for this tree in the local Gubbi Gubbi language).
Although the mini protein is unique, its weird folding pattern or shape is similar to toxins found in some spiders and in cone snails. Another term for this molecular shape is “inhibitor cystine knot” (or aptly ICK or knottin). Apparently, the amino acid chain folds in on itself a couple of times, and sulphur atoms in one amino acid link up with another amino acid to hold the structure in a tight knot.5
The action of the gympietides (the knot) involves its victim’s nerves. If you recall your high school biology you’ll remember that the transmission of a signal along a nerve involves sodium ion gates that open in the nerve cell membrane allowing sodium to rush into the nerve cell. As the signal proceeds down the nerve cell, the previously opened gates slam shut so that the cell can return to its former condition in preparation for receiving a new signal.
What the gympietide poison does is open the sodium gates and then doesn’t allow them to close or recover. Thus the scientific team reports that:
“The intense pain sensations and [nerve cell] reflex flare observed after [poisoning] by Dendrocnide species are consistent with the potent activity of the gympietides at [sodium voltage] channels [in the nerve cell membrane].”6
While the order of amino acids in the protein chain from the stinging tree’s toxin has not been observed anywhere else, nevertheless the folding pattern confers on the molecule an effect similar to some spider and cone snail toxins.7 Thus the study authors conclude concerning the gympietides:
“Their structural similarity and a delivery mode identifiable as envenomation exemplify cross-kingdom convergence of venoms.”8
The scientists can scarcely contain their surprise when they reflect that these close similarities in design are found between members of different kingdoms. Of course, plants and animals could scarcely be more different from each other in appearance, capabilities, and lifestyle needs. Whatever could lead to evolutionary processes which start so far apart but end up with a product so similar?
As to whether there could be an evolutionary reason for a plant to produce animal venoms, the scientists declare that the issue remains “unclear.”9 Indeed it seems obvious that an evolutionary answer will never be found. Rather, the explanation is clearly that these were choices made by God. In our fallen world there are many agents of death and disease. That is not how it was supposed to be. Nevertheless, these agents demonstrate the same intricate characteristics as the rest of the Creation.
Whale and shrub
If similar compounds produced by a tropical tree and a marine snail are difficult to explain from an evolutionary point of view, how about liquid waxes from a whale and a desert shrub?
According to an article from University of Washington Magazine, up to 1972 when the Endangered Species Act was passed in the United States, in North America alone up to 55 million pounds of sperm whale oil were used to protect automobile transmissions. According to the article, thanks to protection from whale oil, prior to 1972, car transmissions seldom failed. Within three years of the moratorium on whale killings, the rate of car transmission failures in the US increased 800%. Thus, the article declares: “Because the automatic transmission is the second-most expensive component in a car and the most complex to repair, total sales for transmission shops exceeded $50 billion by the 1990s.”10 The problem is that the sperm whale liquid wax was just the right product to provide for excellent lubrication in car transmissions and there was no other similar product available.
The oil of the sperm whale Physeter macrocephalus is a liquid wax. The characteristics which made this product so perfect for lubrication included the following. For a start and most uniquely, this wax is liquid at room temperatures. Also, it is viscous (much thicker than water) but slippery and not sticky. And most importantly, this viscosity does not change much with greatly increased temperature and pressure such as we see in running motors. For example, if you were expecting a product to lubricate your engine, but the product became much more fluid with increasing temperature and pressure, your engine would soon seize up. Also helpful are the facts that liquid wax does not readily oxidize (breakdown) and it flows in cold weather rather than congealing.
Deeply concerned that they had lost an exclusive and useful product, the automobile industry began desperately to search for alternatives. And they soon found one in seeds of a desert shrub, the Simmondsia chinensis, or jojoba. A professional oil chemists’ journal in 1979 declared: “The protected but still endangered sperm whale and desert-grown nuts from jojoba are the only major sources of liquid waxes.”11 Similarly, an article declared in 2009: “Jojoba oil is very similar to that of spermaceti for which it is an excellent substitute.”12 Later in 2017 scientists writing in Biological Research describe jojoba oil as a “high-viscosity liquid-oil that differs from any other oil produced by plants”13 so that “The jojoba oil plant is a promising alternative to threatened sperm whale oil.”14 So, the world did an about-face and focused their attention on the desert instead of the sea.
Slick similarity
So why are these oils, from two such different sources, so similar and otherwise so unusual? The secret of these oils is their chemical identity as liquid waxes. Without embarking on a crash course in organic chemistry, we find that most organic oils are fats. Fats involve long chain fatty acids linking up with a glycerol molecule. Glycerol has only three carbon atoms, but each of them is usually connected with a long chain fatty acid. This makes quite a complicated molecule, like a glove with three very long fingers.
Liquid waxes are totally different. A moderately short chain fatty acid links with a similar molecule which ends with an alcohol grouping instead of an acid. So, we just have one straight chain of carbons in a liquid wax. For whale oil liquid waxes, we generally see 28 to 32 carbons.15 As organic compounds go, these are small molecules. For jojoba, the liquid waxes are a little longer, from 38 carbons to 46 carbons.16
The commercial exploitation of jojoba liquid waxes is not totally straightforward. The oil is found in the seeds (up to 50% by weight), but less than half of the shrubs actually produce seeds. For some reason, there seems to be a bias to grow more male plants than female plants and one cannot identify the female plants until they flower, several years after germination. Although the plants tolerate quite terrible desert growing conditions, the flowers don’t always set seed well. Altogether jojoba liquid is very expensive to produce. Nowadays we see mostly synthetic products of jojoba oil for automotive uses.
The intriguing issue is why two such different organisms happen to exhibit this highly unusual chemistry. Evolutionists would say that this capability came about by chance. Since no other organisms display this capability, it is obvious that these choices were not a case of the organisms needing these waxes for survival. It appears that the liquid wax does enhance germination of the jojoba seeds. Of course, whales don’t care about that. Several explanations have been proposed to explain the large amount of liquid wax in sperm whale heads. There certainly was no common condition encouraging the development of an unusual chemical product in these two creatures.
We see rather God’s whimsical choices in conferring this valuable product on two such different creatures. At this point it seems appropriate to give thanks for the fascinating beauty that we see among living creatures of all types. We also see that diversity and unexpected complexity confer a richness on the Creation which never ceases to comfort us that God is in control.
Dr. Margaret Helder is the President of the Creation Science Association of Alberta which has just published an intriguing new book called “Wonderful and Bizarre Life forms in Creation” which you can learn more about and order by clicking here.
Endnotes
- Edward K. Gilding et al. Neurotoxic peptides from the venom of the giant Australian stinging tree. Science Advances 6: September 16 pp. 1-9. See p. 1.
- Adeel Mustafa et al. Stinging hair morphology and wall biomineralization across five plant families. American Journal of Botany 105 (7): 1109-1122. See p. 1115.
- Mustafa et al. 1121.
- Gilding et al. 1.
- For people who like chemistry, the amino acid cysteine ends in a sulphur atom. And the cystine is formed from 2 cysteine residues joined end to end through the sulphur atoms (disulphide bond). Cystine is formed by linking cysteine residues through their sulphur atoms across different parts of the loop. In a knot, there are two cystine molecules connecting different parts of the chain and another in a different direction which ensures that the knot does not fall apart.]
- Gilding et al. 5.
- Gilding et al. 5
- Gilding et al. 5
- Gilding et al. 5
- Jon Marmor. 2019. The Innovation File: Solving a Whale of a Problem. UW Magazine 1-5. See p. 2.
- K. Miwa and J. A. Rothfus. 1979. Extreme-Pressure Lubricant Tests on Jojoba and Sperm Whale Oils. Journal American Oil Chemists’ Society 56 #8 pp. 765-770. See p. 765.
- Vijayakumar et al. 2009. Synthesis of ester components of spermaceti and a jojoba oil analogue. Indian Journal of Oil Technology 16 pp. 377-381 September. See p. 377.
- Jameel R. Al-Obaidi et al. A review of plant importance, biotechnological aspects and cultivation challenges of jojoba plant. Biological Research 50:25. pp. 1-5. See p. 1.
- Al-Obaidi et al. 3.
- Vijayakumar et al. 377.
- Rogers E. Harry-O’Kura et al. Physical Characteristics of Tetrahydroxy and Acylated Derivatives of Jojoba liquid Wax in Lubricant Applications. Journal of Analytical Methods in Chemistry. 2018 Article ID 7548327 pp. 12. See p. 1.